Accelerator Mass Spectromety Facility
Ion Source
In order to produce an ion beam, an ion source is required. There are several types of ion sources, however, for the AMS experiments at the MLL, Cesium-Sputter sources of Middleton-Type with a sperical ionizer are used. You can see a sketch of such a source in the picture below.
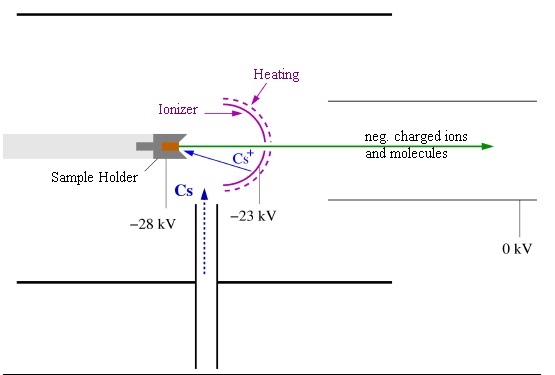
The sample material for the AMS experiment (typically few 10 mg) is placed in a sample holder and inserted into the source from the left on a water-cooled holding rod. Now we require a process that can remove particles from the sample and inject this beam into the beamline and finally into the tandem accelerator.
A reservoir of Cesium is located beneath the source. It can be heated to introduce some of the cesium into the source above. The Cs atoms are then ionized on a tantalum surface which is heated to roughly 1300 K. Tantalum is used because it can withstand the heat due to its high melting point (3290 K). The positively charged Cs ions are then accelerated towards the sample by applying a 5 kV potential. The kinetic energy of the Cs ions is sufficient to eject particles from the surface of the sample. This is referred to as sputtering. At this point, it is important to note that not only single atoms can be ejected from the sample in the sputtering process, but also molecules. These can either be sputtered as a whole or form after sputtering. While sputtered neutral atoms and molecules may leave the ion source and are lost for the experiment, reducing the efficiency of the source, positively charged particles are trapped inside the sample holder due to the same electrostatic potential that accelerates the cesium ions towards the sample. The required beam of negatively charged ions, for example (or molecular ions) is created as follows: Cesium sets down on the sample surface where the temperature is lower due to the water cooling of the holding rod. Sputtered atoms and molecules have to pass this layer of cesium where a charge transfer can occur because of cesium's low electronegativity. The negatively charged ions and molecular ions created in this way have the charge state -1; higher negative charge states are not formed. They are then accelerated out of the ion source with a 23 kV extraction voltage. Typical currents that can be achieved with this source are on the order of few micro-amperes, that means, roughly 10 trillion particles per second. This high current is key when using AMS to scan for smallest traces of radioisotopes, since more examined particles means more sensitivity.
Tandem Accelerator
The facility is equipped with a 14MV MP Tandem Van-de-Graff accelerator. The 25 m long tank contains 7 bar sulfur hexafluoride serving as insulating gas. The negative ions enter the tandem accelerator and are accelerated towards the high-voltage terminal in the center, which is at positive potential (typically 8-12 MV). They then pass through a foil stripper. This is a thin carbon foil (only few micrometers). When ions pass through it, several electrons are removed. Bohr's atomic model gives the rudimentary prediction, that any electron with an orbital velocity smaller than the velocity of the ion will be stripped off. In reality, however, ions with lots of different positice charge states are formed. A very important feature of this stripping process is the suppression of any molecular background. When a molecular ion passes through the foil, the delicate equilibrium between the binding electrons and the electrostatic repulsion of the nuclei is disturbed by the removal of electrons. This causes the molecular structure to break apart, this is also known as a Coulomb explosion.
Since the ions are now positively charged, they are accelerated further by the same potential towards the ground at the other side of the tandem accelerator. The energy to which the ions can be accelerated depends the charge state that is used. However, energies on the order of 100 MeV are typical.
Detection Systems
In order to detect smallest traces of a radioisotope in the sample material, the individual particles in the ion beam have to be identified.
-
Velocity: The ions' velocity can be obtained by measuring the time it takes them to travel a known distance. This is called a Time-Of-Flight (TOF) measurement. As a start signal, Micro-Channel-Plate (MCP) detectors are used. The ions pass through very thin carbon foils (few micrometers) and eject several electrons from them. These electrons are then amplified in the MCPs such that they produce a detectable signal. For a stop signal, e.g. another MCP detector can be used, but there are other possibilities.
-
Energy Loss: The AMS setups feature ionization chambers. They are filled with a few mbars of isobutane gas and contain a capacitor. When an ion passes through the chamber, it ionizes the gas in its path. The electrons created in this way drift to the anode of the capacitor. In this way, the energy loss of the ion in the gas can be measured.
-
Total Energy: To obtain the total kinetic energy of the ions, they need to be stopped. This can be done with a silicon surface barrier detector. In such a semiconductor detector, the kinetic energy of the ion is converted into electron-hole pairs. This signal can also be used as a TOF stop signal.
-
Isobar Suppression: A very important challenge of AMS is separating the desired radioisotope from other isotopes with the same mass (isobars). This can be achieved by using the Gas-filled Analyzing Magnet Setup (GAMS). The GAMS is a dipole magnet filled with few mbars of nitrogen gas. When the ions pass through the gas, they change their charge state due to the interactions with the gas. This change depends on the atomic number Z of the isotopes. The isobars now have different charge state distributions and thus different radii passing through the magnet which results in a spatial separation of the isobars.