Doppler Shift Lifetime Station
Reactions in explosive stellar events involve proton capture into excited nuclear states of the new nucleus thus formed. For example, we have a schematic astrophysical reaction like the following: X + p → Y* → Y + γ. The asterisk denotes that nucleus Y is formed in an excited nuclear state, which then promptly de-excites by gamma-ray emission, to the ground state of Y. We typically abbreviate this notation in the form: X(p,γ)Y; everything to the left of the comma is on the left side of the arrow in the original equatin, and similarly for everything to the right side of the comma. It is the rates of such reactions, under the astrophysical conditions within nova or x-ray burst explosions, that we seek to measure. An important nuclear physics quantity that is essential to determine this rate is the lifetime of the excited nuclear states in Y into which the proton is captured.
In our experiments, we populate the astrophysically important excited states in Y with a transfer reaction, schematically represented as: Z + a → Y* + b. Nucleus "a" for example, could be a deuteron, 3He, or an α particle. Nucleus "b" is called the transfer ejectile; it, too is a light nucleus, such as a proton, deuteron, 3He, or an α particle. (a and b are, of course, different). Once again, the excited nuclear state in Y promptly decays by gamma-ray emission.
By measuring the ejectile particle "b" in coincidence with the gamma-rays from Y*, the lifetime of the excited nuclear states can be determined.
The Method
The method of the measurement is as follows. A target foil with high stopping power (such as gold) iis first implanted with the light target nuclei, such as 3He or α particles. These implanted nuclei are what we call particle "a" in the schematic reactions above. The gold foils are approximately 15 microns thick, and only the first 0.5 microns of thickness are implanted with the light nuclei "a". A beam of accelerated nuclei from our tandem accelerator is then directed directed onto this target foil and transfer reactions between the beam nucluei and the implanted nuclei occur. When this happens, we produce the nucleus Y in one of its excited states, somewhere in the first 0.5 microns of the target foil, along with the light ejectile particle designate "b" above.
The animation below illustrates the process described above.
The excited nuclear state has a finite lifetime, albeit very short. The excited nucleus Y* undergoes its decay via gamma-ray emission, and does so while in motion and while slowing down in the target foil. A high purity, large volume, germanium gamma-ray detector detects the gamma-rays from the excited state decays. The gamma-rays will be Doppler shifted, since Y* decayed while in motion. However, not every nucleus Y* that is formed in the experiment will decay with the same velocity and, therefore, the same Doppler energy shift. The reasons fort this are as follows:
- the nuclei are decaying as they are slowing down in the target foil (not in vacuum).
- the probability for the excited state to decay in a given time interval has a decaying exponential time distribution. Not every Y* nucleus decays at exactly the same time after being produced.
For these two reasons, the resulting gamma-ray spectrum recorded by the Ge detector has embedded into it the combination of both the exponential time distribution for the decay of the excited state and the velocity of the nucleus at the instant the excited stated underwent decay. Unfolding these two pieces of embedded information requires knowledge of the stopping power of nucleus Y in the target foil.
Theoretical models of the slowing-down process of Y in the target foil, combined with the exponential lifetime distribution for the excited state, allow the lifetime of the excited state to be extracted from the gamma-ray spectrum recorded by the germanium detector.
The Facility
The facility consists of a target chamber into which is mounted a copper target ladder designed with 6 target holders. Also situated in the target is a movable base onto which up to 3 silicon charged particle detectors can be mounted. Upstream of the target is a copper tube, approximately 45 cm long, and concentrically aligned along the beam axis. This tube has two electrically isolated collimators inside it spaced approximately 30 cm apart; they are used for tuning the beam onto the target. The tube also is in thermal contact with a liquid nitrogen (LN2) reservoir. The end of the tube, closest to the target, has a copper block to which are attached two copper leaf springs. These springs come into contact with the target ladder, when the target ladder is raised into position.
The figure below shows a design diagram of the entire experimental setup.
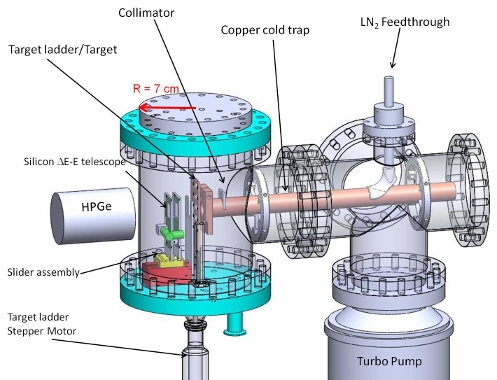
The system can be pumped down to ≈10-7 mbar. The copper tube is cooled with LN2 after the turbo pumps have lowered the pressure in the system to this value. Cooling of the tube causes adsorption of any remaining residual gas molecules onto its surfaces. Only after this do we raise the target ladder into position against the leaf springs for cooling. We cool the target to avoid diffusion of the implanted nuclei under beam heating. Furthermore, the target is raised into contact with the leaf springs after sufficient time has passed to adsorb residual gas onto the copper tube. This is done to avoid buildup of contaminants on the surface of our targets could otherwise react with the beam and be a potential source of unwanted background. Situated outside the target chamber, and capable of rotating ≈ 300° around the chamber, is the germanium gamma-ray detector.
The photo below shows a view looking directly into the target chamber from the top. To the left one sees the copper tube entering the target chamber and the copper block attached to its end. Next, immediately to the right of the copper block is the target ladder. To the right of the target ladder, one can see the three mounting frames that hold our silicon detectors comprising a position sensitive ΔE-E telescope with which we identify the type of ejectile particle (proton, 3He, α, etc) and determine its energy and scattering angle.
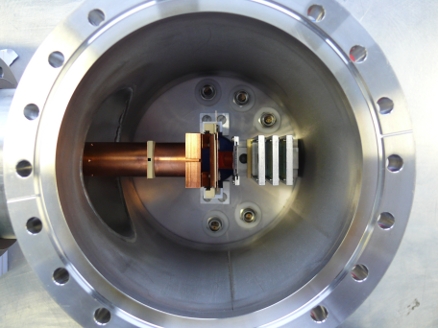